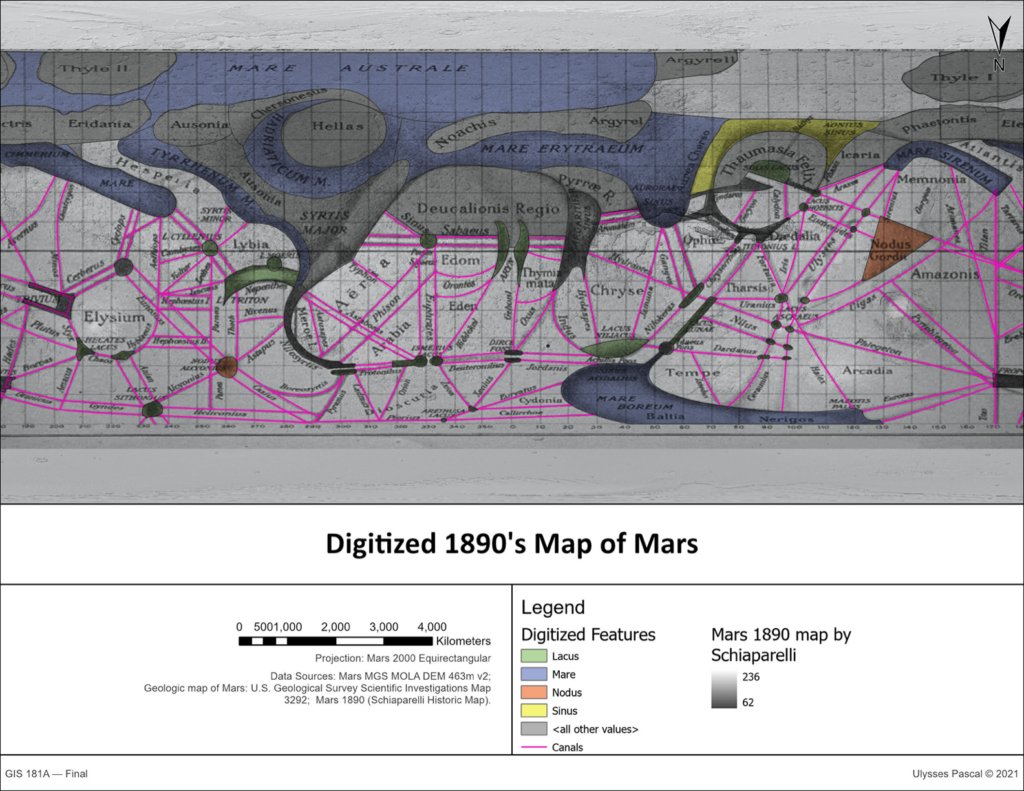
Introduction
The Italian word canale has two senses: 1) geologically formed channels, and 2) artificially constructed canals. In a 19th century letter regarding the possibility of canale on Mars, the Italian astronomer Giovanni Schiaparelli wrote “It is as impossible to doubt their existence, as that of the Rhine on the surface of the earth.”[1] Though Schiaparelli described these canale as “grooves”, his discoveries were mistranslated into English as “canals”.[2] Evidence of the presence of canals on Mars was originally confirmed in 1879 by the American astronomer Percival Lowell.[3] With more advanced techniques, large geologically formed channels have been discovered by the Viking Obiter, but the highly geometric features depicted in the maps made by Schiaparelli and Lowell have not been observed.[4] Nevertheless, the belief in Martian canals inspired generations of science fiction authors, such as H.G. Wells, and shaped our cultural attitudes to the Red planet.[5]
Given the enduring influence that Schiapraelli’s maps have had on our imagination of Mars, how accurate were Schiaparelli’s observations? We can now compare his maps directly against remote sensing data. We can also investigate the possibility of canale in more detail with surface rovers. If we were able to send a rover to investigate Schiaparelli’s canals, what would be a suitable landing site? To answer these questions, this project geo-references Schiaparelli’s 1890 map of Mars and uses satellite imagery of Mars to conduct a suitability analysis of landing sites for a martian rover.
Methods
To determine the optimal location of landing sites on Mars, this project uses suitability analysis. The optimal location for a landing site would be near regions of interest and far from potential dangers. Additionally, the optimal landing site should not be close to existing rover sites in order to reduce the redundancy of rovers on Mars. The regions of interest for this mission are near Schiaparelli’s canale. Potential hazards that could jeopardize the landing of a rover are 1) steep incline 2) dust storms and 3) rough terrain.[6] To prepare the data for suitability analysis, this project uses geo-referencing, digitization, and map algebra.
To georeference the Mars 1890 Schiaparelli map, I created a spreadsheet of every intersection point on the latitude and longitude grid that is present in the original map (n=518). Using the coordinate points along the edges and center of the map and a third order transformation, I aligned the Mars 1890 map to the grid of points. I used the remaining points to test for precision. This procedure resulted in a transformation with a root mean squared error (RMSE) of 0.413. I then visually inspected the map and the underlying hillshade raster to validate that known features in the Schiaparelli map correspond with the satellite data.
Once georeferenced, the map was digitized using polygons for large regional features and lines for the canale. To prepare the data for suitability analysis, I created 10 ring buffers around the canale at 10km intervals and then rasterized the layer.
Rank | Layer | Function | Weight | Rationale | Max |
1 | Canali Buffer | MSSmall | 3 | Must be close to a feature of interest. | 30 |
2 | Slope | MSSmall | 2.5 | Must avoid dangerous terrain. | 25 |
3 | Relief (standard deviation of DEM) | MSSmall | 2 | Must avoid difficult to navigate terrain. | 20 |
4 | Dust storm Density | Small | 1.5 | Should avoid hazardous conditions. | 15 |
5 | Distance from previous landing sites | Power | 1 | Should avoid prior explorations. | 10 |
SUM: | 100 |
To quantify the hazards, I performed slope analysis and relief analysis on a DEM of Mars. To calculate the relief, I used the standard deviation of the elevation, which gives a measure of the roughness of the terrain. To estimate the danger of dust storms, I computed the kernel density of sandstorms from dust storm point data (n=14974). I reclassified the dust storm kernel density on a 1 to 10 scale, with the lowest density of dust storms ranked as the most suitable for a landing site. Last, I created a dataset of landing sites between 1971 and 2021 using data from NASA.[7] I used the geodesic distance from each landing site to determine regions distant from existing landing sites.
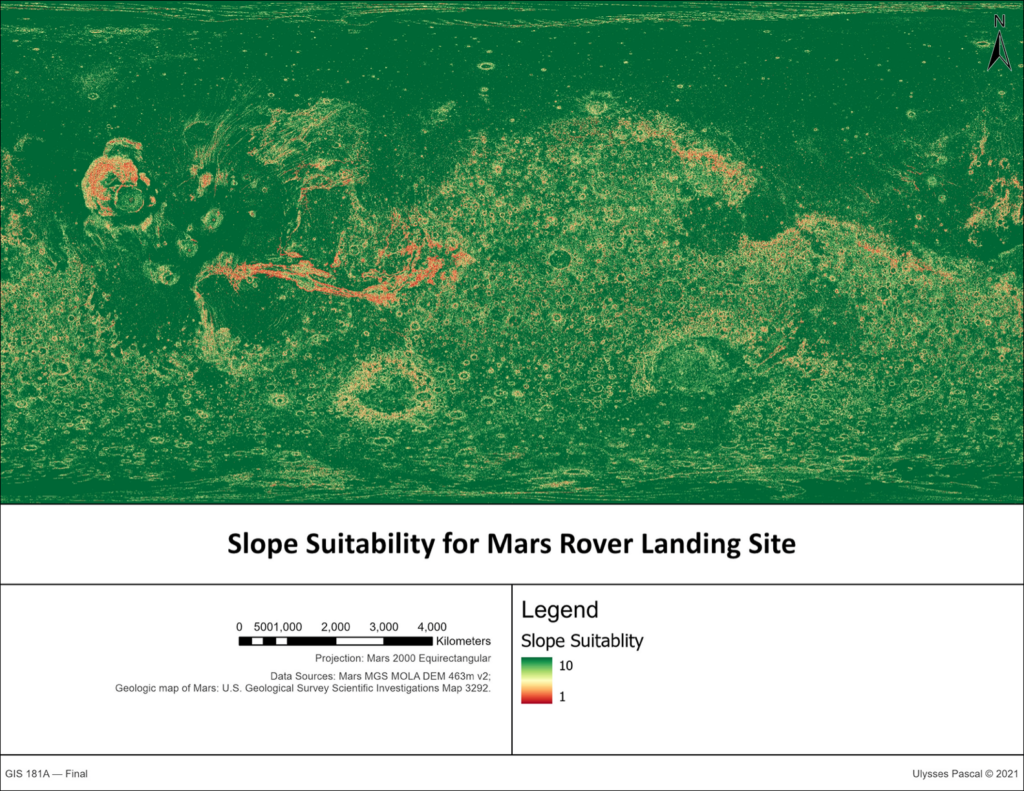
Results
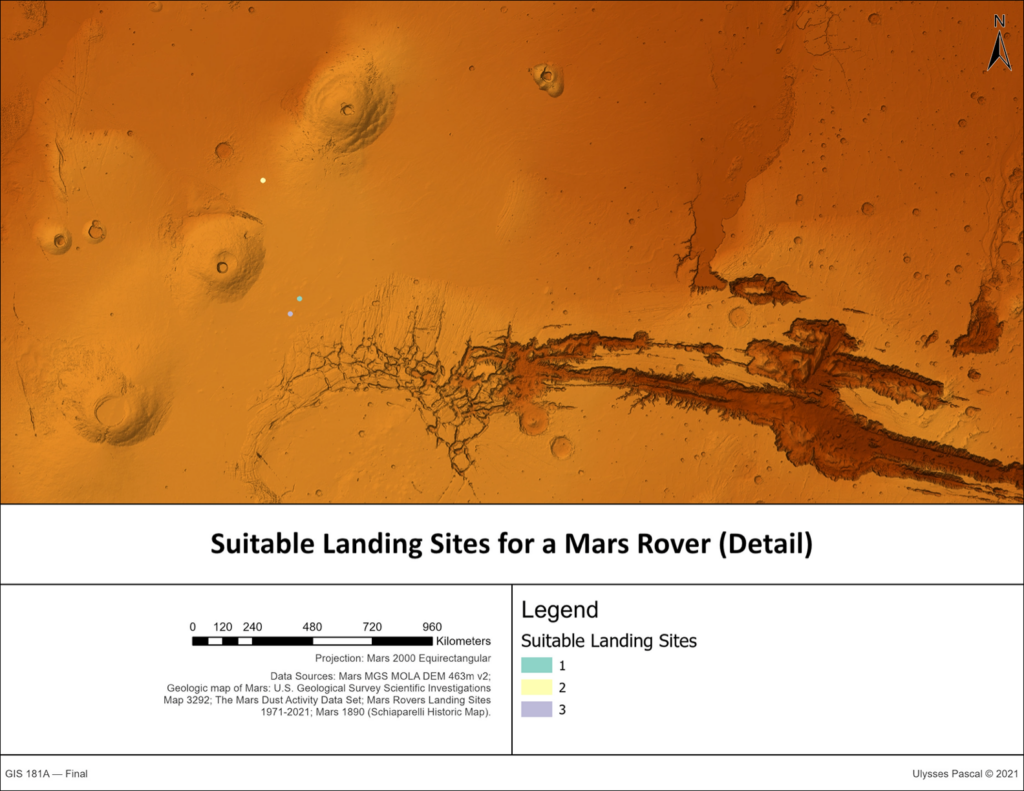
From the digitization procedure, it is evident that toponyms from Schiaparelli’s map are still used to describe features in modern maps, however few actual geological features correspond with features in Schiaparelli’s map. Names of broad regions such as Elysium, Hesperia, Stryis Major, Acidalia Planitia, Tempe Terra, Amazonis Planitia, and Arcadia Planitia persist in contemporary maps but these are not names of discrete geological features. Only the largest geological features, such as Hellas crater, correspond with remote sensing data. Of these, only one canale network in Schaiparelli’s map corresponds with the data: the Valles Marineris. This valley is Mars’ largest valley. In fact, NASA reports that it is the “largest valley in the solar system.”[8] However, the numerous straight-line canale that mark Schiaparelli’s map do not exist in maps derived from digital elevation data.
If a there were a mission to send a rover to Mars to explore the possibility of Schiaparelli’s canale, the most suitable locations would be in the northern hemisphere of Mars, as this region of Mars has the least variability in elevation and it corresponds with a region in Schiaparelli’s map that contains several canale. The suitability analysis model was successfully able to locate three landing sites within 10 km of a canali that are distant from hazards and prior Mars rover landing sites.
Limitations
This project was limited by two factors: uncertainty and computational complexity. The quality of the Schiaparelli map is a source of uncertainty because of its low fidelity and because the scanned resolution is low given the level of detail that the map contains. These factors produce uncertainty in terms of georeferencing and in terms of transcribing the toponyms. The extremely large region presented computational difficulties that prevented comparing multiple iterations of the suitability model. In future studies, further analysis should be appended to the suitability analysis. There are missing parameters in the suitability analysis which are contingent on the design of the rover and landing device, such optimal reflectance for solar panels and optimal temperature for the functioning of components. A more robust measure of dust storm probability could be developed with more advanced statistical techniques. Lastly, if canale were actual grooves on the surface of Mars, the model parameters of slope and relief may be biasing the model against locations of real geological channels. To find landing sites near actual channels, such as the Valles Marineris, the weight of these parameters should be lowered.
Conclusion/Discussion
Schiaparelli’s impact on our orientation towards Mars is not limited to science fiction. Several toponyms from Schiaparelli’s 19th century research persist in contemporary maps. However, many of the actual geological features—most noticeably the canale—are not present on Mars except for Valles Marineris, which appears both in Schiaparelli’s maps and in satellite imagery. This is not surprising, as Valles Marineris the largest valley in the solar system. If Schiaparelli’s canale were to be investigated by a rover on Mars, there are several suitable locations that take into account proximity to canale, hazards, and existing missions on Mars.
References
[4] Baker, V. R.. The channels and valleys of Mars. Third International Colloquium on Mars. Aug 31-Sept 2, 1981. https://articles.adsabs.harvard.edu//full/1981LPICo.441…12B/0000012.000.html. (Accessed December 4, 2021).
[2][5] Johnson, William B., and Thomas D. Clareson. 1964. The Interplay of Science and Fiction: The Canals of Mars. Extrapolation 5, (2) (May 01): 37.
[1] Green, Nath. Mars and the Schiaparelli Canals. The Observatory.
[3] Manara A. and A. Wolter. Mars in the Schiaparelli−Lowell letters. Mem. S.A.It. Vol. 82, pp. 276-279. https://adsabs.harvard.edu/pdf/2011MmSAI..82..276M. (Accessed December 4, 2021).
[8] NASA. Valles Marineris: The Grand Canyon of Mars. https://www.nasa.gov/multimedia/imagegallery/image_feature_83.html. Mar 23, 2008. (accessed December 4, 2021).
[6] Pingyuan Cui, Dantong Ge, Ai Gao. Optimal landing site selection based on safety index during planetary descent. Acta Astronautica. Volume 132, 2017. Pages 326-336.
[7] Tanaka, K.L., Skinner, J.A., Jr., Dohm, J.M., Irwin, R.P., III, Kolb, E.J., Fortezzo, C.M., Platz, T., Michael, G.G., and Hare, T.M., 2014, Geologic map of Mars: U.S. Geological Survey Scientific Investigations Map 3292, scale 1:20,000,000, pamphlet 43, https://pubs.usgs.gov/sim/3292/. (Accessed December 4, 2021).
Data Sources
MOLA Team. Mars MGS MOLA DEM 463m v2. Goddard Space Flight Center. Jan. 13 2014 – Feb. 3 2020. https://astrogeology.usgs.gov/search/details/Mars/GlobalSurveyor/MOLA/Mars_MGS_MOLA_DEM_mosaic_global_463m/cub. (Accessed December 4, 2021).
Michael Battalio, Huiqun Wang. The Mars Dust Activity Database (MDAD): A comprehensive statistical study of dust storm sequences. Icarus, Volume 354, 2021. https://doi.org/10.1016/j.icarus.2020.114059
The Mars Dust Activity Data Set. Harvard Dataverse. URL: https://dataverse.harvard.edu/dataset.xhtml?persistentId=doi:10.7910/DVN/F8R2JX. (Accessed December 4, 2021).